Key Biosafety Considerations for Coronavirus Research
The recent outbreak of the novel 2019 coronavirus (2019-nCov) in Wuhan, Hubei Province, China, has led to a surge of interest in coronavirus research and concern about the risks associated with coronaviruses. In this blog we’ll introduce coronaviruses and cover strategies for developing medical countermeasures to combat the recent outbreak. We’ll also review opportunities, risks, and risk mitigation strategies for coronavirus research.
Coronavirus (CoV) is a family of viruses that cause disease in mammals and birds. Most coronaviruses capable of infecting humans cause mild to moderate respiratory tract infections which are transmitted by respiratory droplets produced when an infected person coughs or sneezes. Certain notable coronavirus species can cause more virulent infections, including SARS, MERS, and 2019-nCov.
Over the last few days the nomenclature for this virus has changed: the World Health Organization (WHO) named the pneumonia associated with the infection “Covid-19 (coronavirus infectious disease 19),” and the International Committee on Taxonomy of Viruses named it “severe acute respiratory syndrome associated coronavirus 2 (SARS-CoV-2)” due to the virus’ genetic similarity to the SARS associated coronavirus (SARS-CoV) responsible for the 2003 outbreak that caused 8098 infections and 774 deaths. For simplicity, in this article we’ll refer to the virus as 2019-nCoV and the pneumonia associated with the infection as Covid-19.
An important early victory in the fight against the 2019-nCoV is the sequencing of the viral genome, which has led to genetic tests/molecular diagnostics allowing for confirmation of infected individuals. The viral genome was originally made available by the Chinese government, and the CDC is making the sequences of confirmed US cases available online. The CDC has also made molecular diagnostic kits available to authorized labs so that a suspected case can be confirmed or ruled out within hours, rather than waiting days for samples to be shipped to the CDC for processing at a central lab.
Strategies for developing medical countermeasures
Antivirals and antibody-based treatments
Previously developed antivirals are actively being tested in the laboratory setting to evaluate if they can impede 2019-nCoV replication in cultured cells. Early tests show remdesivir and chloroquine may be effective, and the first US Covid-19 case was successfully treated utilizing remdesivir under a compassionate use protocol. Several clinical trials are currently being initiated to assess the safety and efficacy of various antiviral treatment regimens, including two Phase 3 trials testing remdesivir on Covid-19 patients in China.
Antibody-based treatments, which can bind to the virus in infected individuals and allow the immune system to destroy the bound virus particles, are also being investigated. Such passive immunization with neutralizing antibodies would confer transient protection to patients in a critical disease state—much like an anti-venom following a snake bite—allowing patients to recover from a critical disease state and for their immune system to clear the infection. Fortunately, the genetic similarity between SARS-CoV and 2019-nCoV suggests there is potential that antibodies developed against the earlier outbreak may be effective against the current one. One biotechnology firm claims to already possess two antibodies developed against SARS-CoV that bind to 2019-nCoV.
Vaccines
While antivirals and antibodies can be utilized to treat the disease, vaccines are the best way to prevent viral infection and control outbreaks. An effective vaccine can create an immune population no longer susceptible to infection and prevents the virus from spreading. A ring of vaccination around an outbreak can contain it and allow it to burn itself out without spreading to new hosts. Several groups are currently developing vaccines against 2019-nCoV, including pharmaceutical companies and the NIH.
Vaccines require two main components: a target antigen for the immune system to attack and an adjuvant to spur the immune response. The primary target for a vaccine is the spike protein on the virus surface, which the virus utilizes to bind to and infect host cells (see below). Antibodies binding to the spike protein prevent the virus from infecting host cells and label it for destruction by the immune system.
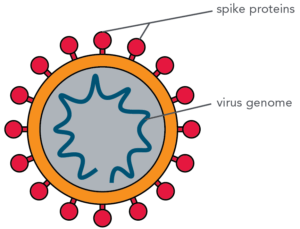
Various design strategies exist to achieve these objectives. Modern vaccines typically involve an inactive or attenuated form of the organism being vaccinated against (e.g., oral or inactivated polio vaccines, MMR vaccine) or a subunit vaccine containing only the antigenic parts of the organism (e.g., hepatitis B virus vaccine, gardasil human papillomavirus vaccine).
Currently available genetic engineering techniques and the 2019-nCoV genome sequence provide opportunities for research and development of new vaccines. Scientists can easily introduce single genes from the 2019-nCoV into expression systems to produce subunit vaccines. Introducing 2019-nCoV genes into weakened vaccine strains of existing viruses also serves as a means of delivering both the 2019-nCoV antigen and an adjuvant to spur the immune response.
Minimizing Risks Associated with Researching Genetically Engineered Vaccines
There are risks when utilizing genetic engineering technology. Introducing a coronavirus gene into another virus may have unintended consequences and pose occupational safety risks, as well as risks to the community and environment surrounding the research site. It is also important to mention that using the complete genetic sequence can enable creation of the virus in the laboratory setting. While a typical Biosafety Level 2 (BSL-2) laboratory provides adequate containment for expression of subunits, Biosafety Level 3 (BSL-3) containment is required for culturing and characterizing the virus as well as assaying vaccine efficacy in a challenge model utilizing laboratory animals.
BSL-3 laboratories are designed to contain aerosol transmissible pathogens. Lab design criteria include a double door entry system to provide security and an anteroom for donning and doffing personal protective equipment (PPE) including respiratory protection. The laboratory must have unidirectional, inward flowing, single pass air that is exhausted through a HEPA filter. All work must be performed within a negative pressured and HEPA filtered biosafety cabinet or another aerosol containment device. The facility must contain an autoclave for sterilizing infectious waste.
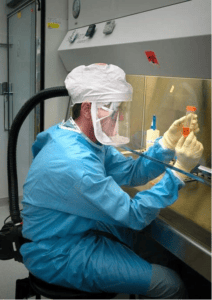
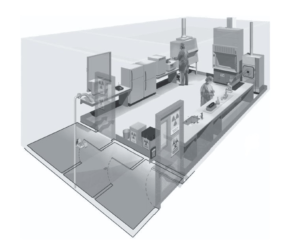
The role of an institutional biosafety committee (IBC) is to conduct risk assessments for research involving engineered genetic material to ensure an appropriate risk mitigation plan is in place. IBC review is required when the research or the site/institution has ties to NIH funding. Even if there are no ties to NIH funding, IBC review is considered a best practice, and NIH recommends voluntary compliance.
Institutions embarking on coronavirus research should consult IBCs with adequate expertise to understand the science, risks, necessary safety practices, and regulatory requirements. There may be additional regulatory constraints depending on the coronavirus species: for example, since 2012 SARS coronavirus has been tightly regulated by the CDC as a select agent for its ability to pose a severe threat to public health and safety.
Time is of the essence in research involving an emergent infectious disease. IBCs at Institutions meet monthly or quarterly, and obtaining approval may take several review cycles. A centralized commercial IBC may be better equipped to conduct reviews efficiently: for example, in 2019 Advarra’s central IBC service has averaged turnaround times of about seven business days from submission to review.
Past research and current gene editing technology provide valuable tools for responding efficiently and effectively to this new coronavirus outbreak. In this kind of fast-paced environment, appropriately managing the risks associated with genetically engineered viruses is critical to developing treatments safely and responsibly.
Need support with your upcoming infectious disease study? Not sure where to start with biosafety measures? The experts at Advarra have worked in a variety of institutional and industry settings (including overseeing BSL-3 labs) and can help you address human subject protection, biosafety, and other research compliance needs. Contact us to get started.